The Orphans of PS1: deserted and detonating
February 23rd, 2011 by mattmccrum Tags: Pan-STARRS1, supernovaStars, such as our Sun, may appear to be ever-present sources of heat and light, but they are highly dynamic objects which will eventually reach the end of their evolutionary paths and die. Although the death of the Sun will be comparatively quiet, more massive stars (with masses greater than 8 times that of the Sun) can be ripped apart when their iron core collapses to an extremely dense stellar remnant known as a neutron star and a shock wave driven by the production of a huge number of particles called neutrinos blasts away the outer layers of material in a supernova explosion. This ejected material does not go to waste however and the elements created, such as carbon and oxygen, provide the Universe with the building blocks it needs for the formation of rocky planets like Earth and all living things upon it.
The process outlined above will lead to only one of the different types of known supernovae, called core-collapse supernovae. These core-collapse type events can then be further divided into sub-classes (type Ib/c, type II) depending on the mass of the progenitor star which explodes. One of the most luminous type II explosions was recorded at 100 billion times the brightness of the Sun and could easily be identified despite being 4.7 billion lightyears away. A second type, a thermonuclear supernova (type Ia), is thought to occur when a dead remnant of a star like the Sun known as a white dwarf star which happens to be in a close binary system accretes matter from its companion, pushing it past its upper mass limit (the Chandrasekhar limit) of 1.4 solar masses. In the last two years incredibly bright supernovae (100 times brighter than an average SNe) have been discovered which may require a new physical explanation for their luminosity and observations from the Pan-STARRS 1 survey (PS1) will help further our understanding of these ultra-luminous events and better define the very interesting physics which leads to them.
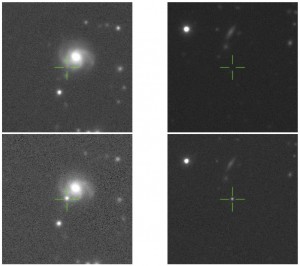
Pre-supernova (top) and post-supernova (bottom) PS1 images showing the discoveries of a supernova with a clear host (left) and a hostless supernova (an orphan, right).
The basic discovery and classification of supernovae is a two-part process. Difference imaging, where nightly images are subtracted from a previously obtained reference image to highlight any objects with a variable flux, is used to detect these very characteristic transients. This is essentially an interstellar spot-the-difference and some examples of PS1 images containing supernovae can be seen in the images to the left. After the initial discovery of a transient that is thought to be a supernovae, follow up spectroscopy must be done in order to confirm the nature of the variable object (it might be simply a variable star or an active galactic nucleus). If it is a supernovae, the spectra can be used to determine elements involved in the explosion and thus deduce its type. By compiling large datasets of confirmed events, the rates of different species of supernovae can be estimated giving us invaluable information on stellar populations within various forms of galaxies and the intergalactic medium.
Up until now, both professional and amateur searches for supernovae have concentrated their efforts on large galaxies with high star formation rates to ensure a large number of successful discoveries. The PS1 survey is not a dedicated search for transients but hosts a multitude of varied science projects meaning that the Hawai’i based, 1.8m telescope, with its 7 square degree field of view focused onto the world’s largest CCD (1.4 gigapixels), surveys massive, unbiased portions of the sky every night. This results in supernovae discoveries in unexpected places as demonstrated in the right hand set of images above. This type of transient is known as an Orphan due to its apparent lack of association with any other object. The object may very well have a host galaxy, but one that is simply too faint to be imaged with the PS1 survey suggesting that it is either at a high redshift or has a low proportion of elements heavier than Helium. If the galaxy has a high redshift (and thus is at a great distance from us) the high luminosity of supernovae events allow us to probe deeper into the universe than the photometric limit of a telescope may at first suggest. If the event occurs within a faint host galaxy, the type of supernovae can give us evidence as to the population in these mysterious stellar neighbourhoods. A possible progenitor channel for the ultra-luminous supernovae mentioned earlier may be pair instability in stars which are quite deficient in the heavy chemical elements (like carbon and all heavier elements). Previous searches in relatively nearby, metal rich galaxies have therefore missed these exiting and currently obscure phenomena.
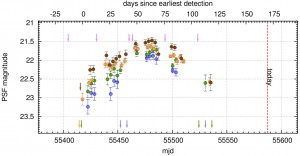
The lightcurve of a hostless SNIIn from PS1 photometry. The different coloured points represent brightness measurements in different filters (g, r, i and z).
Throughout the 3 year period of my PhD at Queen’s University Belfast, under the supervision of Professor Stephen Smartt and Dr. Rubina Kotak, I am hoping to classify as many of the orphans from the PS1 survey as possible, offering statistical analysis and comparison of their rates with current estimates. The PS1 telescope has an r-band photometric limit deeper than magnitude 21 (over one million times fainter than what the human eye can see on a dark night) which is easily capable of detecting these events and producing thorough and defined lightcurves, such as the one shown on the right. Of the almost 250 supernovae with faint or no visible hosts already compiled, at least 3 are thought to fall into the ’ultra-luminous’ category and with hundreds of new transients being detected by PS1 each week, more are sure to follow. With a little luck I will be able to obtain decisive experimental data and perform intense follow-up observations of some of these objects in an attempt to further our understanding of this latest addition to the study of supernovae. Another group, the Palomar Transient Factory, is also looking for these fascinating events (more information on their project can be found here). Possible collaborative work with this team, and some constructive rivalry, will ensure that the science goals presented here are carried out to optimal completeness.