Just keep staring
November 27th, 2012 by ps1scblogThe “Pan” in Pan-STARRS stands for panoramic, but roughly a quarter of the telescope’s time is spent staring at the same ten points in the sky over and over again. These may make up under a quarter of one percent of area of the map of the sky Pan-STARRS1 is making, but they have paid big scientific dividends and have the prospect to produce even more interesting research.
The sky is big, really, really big, it’s the size of almost 200,000 full moons. Pan-STARRS1 is spending about half its time mapping three quarters of this multiple times in different colours of light. However some science goals need more regular observations of patches of the sky. One such goal is looking for exploding stars known as supernovae. If you regularly image a patch of the sky you won’t see exactly the same image over and over again. Sometimes you’ll notice that stars are changing in brightness. These can be because they are pulsating stars which vary slightly in brightness. However there are some stars which are so high in mass that they burn out their fuel quickly and then explode as supernovae. By observing the same part of the sky over and over again, the rapid increase in brightness of supernovae in distant galaxies can be observed. This allows the detection of new supernovae with Pan-STARRS1 which can then be followed up with other telescopes. Have a look at the movie below which shows a supernova detected by Pan-STARRS1.

A short movie of a supernova found in one of the Medium Deep Fields of Pan-STARRS1. Notice there is nothing in the middle at the start but gradually the supernova appears and becomes one of the brightest stars in the field before beginning to fade. Credit: Michael Wood-Vasey/Alisa Rachubo/Alex Damewood/University of Pittsburgh/PS1SC
So far Pan-STARRS1 has detected thousands of probable supernovae, many of them a bit strange. Matt McCrum in Belfast has been looking at supernovae which do not have a clear host galaxy. Laura Chomiuk also wrote a post about some especially strange, extremely bright supernovae. Single exploding stars are not the only sources of supernovae. Binary stars can sometimes be so close that one star will rip matter off the other and suck it on to its own surface. If the star receiving the matter is a white dwarf, there is a limit to how much matter can be dumped on it. Once the white dwarf reaches about 1.4 times the mass of the Sun the physical mechanism that supports the white dwarf against collapse is overpowered. The white dwarf then suffers an cataclysmic collapse which also generates a massive explosion. Because this happens at a specific mass, the brightness of these type of supernovae is approximately the same. Hence these can be used as standard candles to measure distances in the universe. Find this type of supernova and you measure how fast it appears to be receding from us and you can measure how fast the universe is expanding at a particular distance. Build up a large sample and you can work out if the universe is accelerating or decelerating. This is was used in the 1990s to determine that the universe was in-fact accelerating. Pan-STARRS1 is currently building up a sample of this type of supernovae to constrain the expansion history of the universe even more.
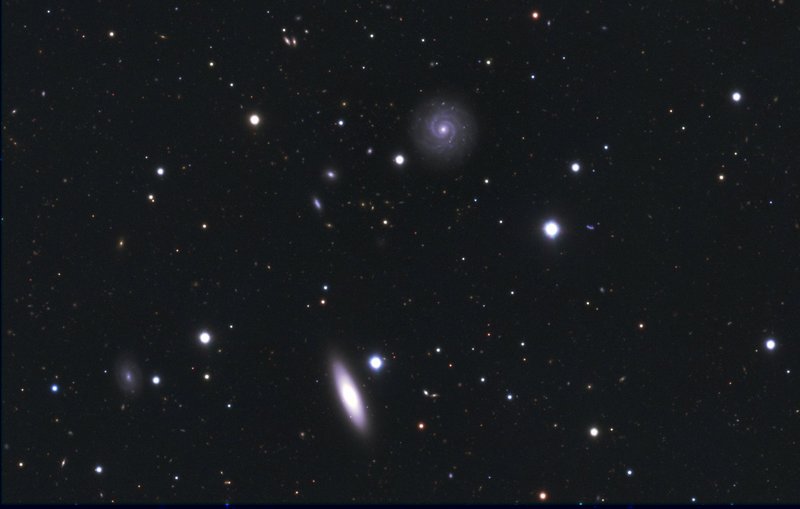
An example of one of the deep, stacked image from one of the Medium Deep Fields. It shows NGC 7684 (lower centre) along with other galaxies. Credit Nigel Metcalfe/PS1SC
Staring at the same place over and over also means you get more and more photons. You can add these up by “stacking” images. While this isn’t literally stacking photographic plates on top of each other, it produces a similar effect, detecting fainter objects than you would from just a single image. Do this with years worth of images over the ten repeatedly surveyed fields (known as Medium Deep Fields) and you get wonderfully deep, detailed images. These fields aren’t chosen at random, they tend to point away from the galaxy so the number of foreground stars is low. Many other surveys have taken data in infrared light as well as the X-ray and radio. By picking areas also covered by these surveys for their deep, repeatedly surveyed fields, Pan-STARRS1 scientists can use these other datasets for studying galaxies in far-flung corners of the universe.
So yes, these fields may be small by Pan-STARRS standards, but the repeated sampling makes them fantastic for characterising parts of the universe that are far away.