MOPS: Finding things that go bump in the night
July 7th, 2011 by larrydenneauYou’ve seen the Hollywood movie version, an astronomer looks through the eyepiece of a telescope (it’s always got an eyepiece), scribbles some calculations on a piece of paper and, with a look of horror flashing across his face, realizes that this is the one, the deadly asteroid that’s going to hit the Earth. In reality discovering asteroids that may threaten the Earth is nowhere near as sensational. But it is somewhat more complicated than and equally as fascinating as the movie version.
Pan-STARRS1’s 7.0 square degree field of view makes it an excellent tool for finding objects moving around our own solar system. Part of PS1’s mission is to discover and catalog these hazardous near-Earth objects (NEOs) and their even more dangerous cousins, potentially-hazardous objects (PHOs). NEOs have orbits that bring them within 0.3 AU (about 45m km) of Earth’s orbit, while PHOs have orbital paths that bring them within 0.05 AU (~7.5m km) of Earth’s orbit and are at least 150m in diameter, large enough to cause extensive damage if one were to collide with the Earth.
To cope with the volume of asteroid data that PS1 and an eventual Pan-STARRS 4 (PS4) would need to handle, the Pan-STARRS project devised its own asteroid-finding software, called MOPS: the Moving Object Processing System. MOPS has been under development for about 6 years, and has proven adept at finding NEOs in Pan-STARRS data and in managing its own catalog of newly discovered and known asteroids beside NEOs so that PS1 scientists can do solar system science.
ASTEROIDS
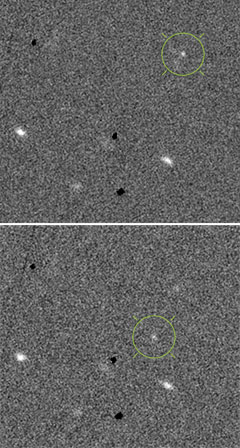
Potentially Hazardous Object ST3 shown moving between two Pan-STARRS images.
By far the largest population of asteroids known lie in the Main Belt between Mars and Jupiter. There are currently about 500,000 known Main Belt Objects (MBOs), a number that increases by a few thousand each month. Occasionally an MBO travels close enough to Jupiter that Jupiter alters the MBO’s orbit so that the MBO transitions to a different orbit. This can be a much more elliptical orbit that sends the MBO well into the inner solar system. If this new orbit brings the MBO close to the Earth’s orbit, it is classified as an NEO. Asteroids range from as large as 950 km in diameter for Ceres (the first asteroid discovered) down to as small as a bus or even a basketball. The smaller asteroids are much more numerous though — while a 1-km asteroid might hit the Earth every million years, a rock the size of a basketball collides with the Earth about once a day.
HOW MOPS FIND ASTEROIDS
Asteroids are first discovered as star-like dots moving between astronomical images taken at the same place on the sky. On short time scales, say less than a day, most asteroids move in a fairly straight line. MOPS uses special spatial-searching software to detect asteroid candidates by playing a large game of dot-to-dot with the millions of star-like sources found in PS1 imagery. PS1′s image processing pipeline (IPP) automatically removes stars, which aren’t moving, so MOPS has the job of trying to find straight-moving combinations of sources in the remaining “transient data” catalogs. We call these nightly associations of asteroid detections tracklets. A large part of the task of finding tracklets is dealing with false sources — star-like image features that come from image artifacts, cosmic rays and random fluctuations in the pixel data. Each night, MOPS scans its transient catalogs for asteroid candidate trackelts, and an IfA scientist confirms real asteroids in each nightly list of candidates.
PS1′s survey designed so that asteroids can be discovered while meeting other science objectives. For example, the PS1 “3π” all-sky survey always obtains images in pairs, so that we can tell if a star-like source is in fact an asteroid because we see it moving between two or more images. About 85% of PS1′s survey time can be used to discover asteroids.
ASTEROID ORBITS
From a single night of observations MOPS cannot determine the complete orbital description of an asteroid. Note that while an asteroid has a straightforward elliptical motion through the solar system, its motion on the sky can be rather complicated due to projection effects. Also, from initial observations we cannot tell how far away an asteroid is from us — we only know its brightness, which can vary according to size and distance. So a faint asteroid might be small or far away; we can’t tell at first.
In order to compute a full six-parameter orbit which describes an asteroid’s motion through the solar system, we need multiple nights of observations of an object, then employ a computational procedure called orbit determination. PS1 uses software provided by NASA’s Jet Propulsion Laboratory — the same software used to guide spacecraft through the rings of Saturn! — and the OrbFit Consortium to fit orbits of solar system bodies to PS1 observations.
FINDING NEOS
Discovering NEOs is even more challenging because they can be found all over the sky, often moving quickly. Unlike main-belt asteroids, which are mostly a similar distance from the sun (2-3 AU) and lie in the plane of the solar system, causing them to appear in a “stripe” on the sky, NEOs can be whizzing by quite close to us and can therefore be projected anywhere on the sky. Repeated PS1 detections of these objects can be quite far apart, and making the dot-to-dot associations more difficult. Because PS1′s survey is largely preprogrammed, PS1 cannot always “chase” fast-moving NEOs to obtain repeated observations. So when we discover a candidate NEO tracklet, we submit the observations to the IAU Minor Planet Center, which maintains lists of NEO candidates that need additional observations. PS1, with its wide field, excels at finding initial observations of new NEOs, but prompt follow-up requires worldwide teamwork and cooperation.
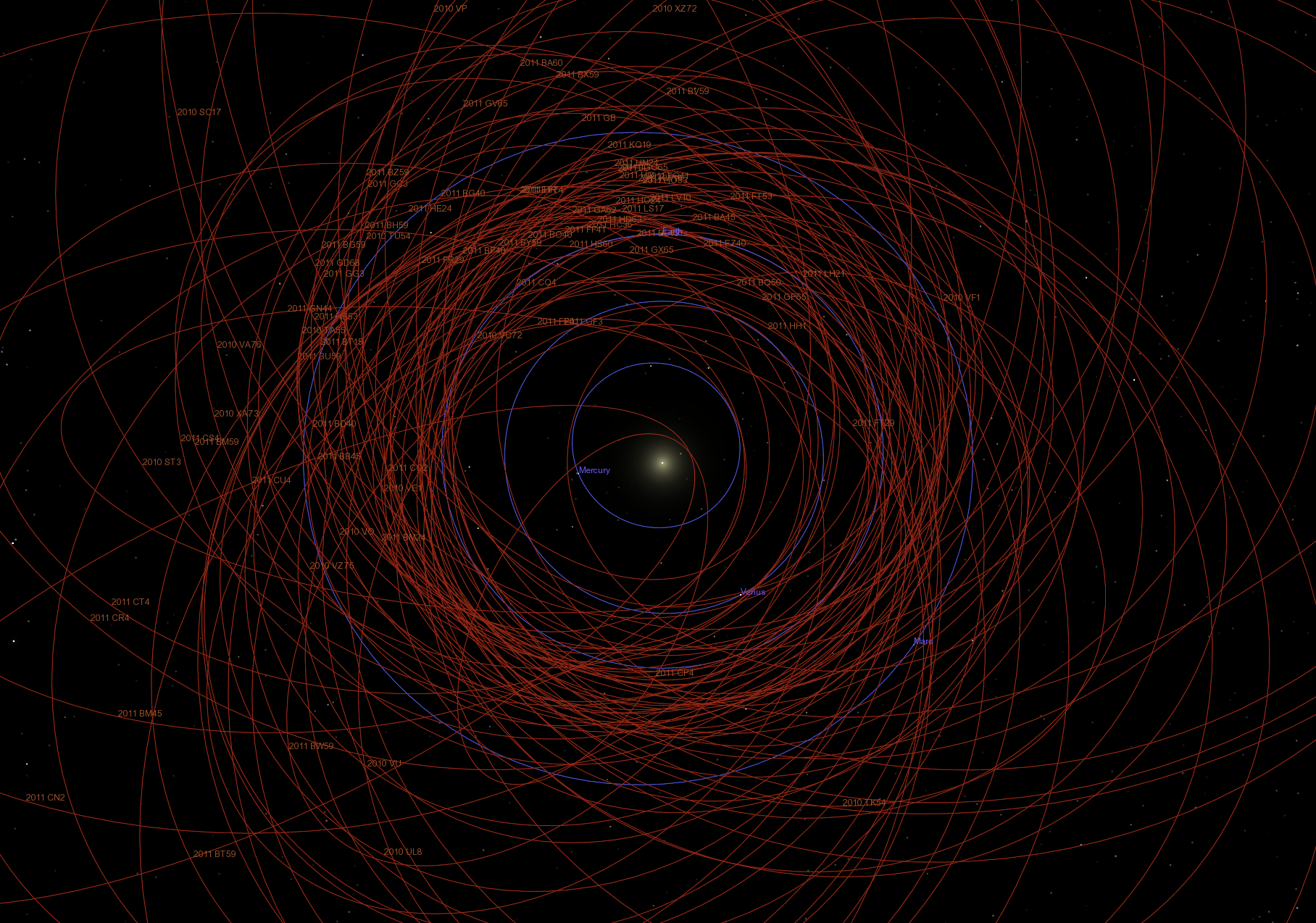
Diagram of the solar system seen from above, showing orbits of NEOs discovered by PS1. Click for large version.
PS1 DISCOVERIES
To date PS1 has discovered 85 new NEOs, two comets, and about 4000 main-belt asteroids. PS1 has also submitted observations for over 200,000 known asteroids — nearly half of all known asteroids! This is an important contribution because PS1′s position measurements are so precise that they substantially improve the accuracy of orbits for known asteroids, allowing us to know their positions even better. Here are some highlights of PS1 discoveries:
2010 ST3. PS1′s first NEO discovery from September 2010.
2011 BT15. An especially hazardous NEO, since we cannot yet rule out an impact in the future between years 2037-2110. JPL maintains a list of still-worrisome asteroids at their risk page.
C/2011 L4. Long-period comet on its way toward the sun from the icy reaches of the outer solar system. This object should be visible to the naked eye in early 2013. PS1SC scientist Richard Wainscoat has more information about C/2011 L4 in another blog post.
OTHER SOLAR SYSTEM SCIENCE
There’s alot more to the solar system than just NEOs and MBOs though. PS1SC scientist Darin Raggozine posted a great summary of outer solar system research, and there’s currently research into newly discovered main-belt comets, “contact binary” asteroids that are fused together, and asteroid impacts. When there’s exciting news to report you can be sure to find it on the PS1SC blog.